Tantti(台湾创新材料股份有限公司)是专业从事研究纳米材料且将纳米球自组装技术商业化的公司,目前领域已经拓展到3D细胞培养支架–Bio-Scaffold。
3D细胞培养支架,又称为生医支架(Bio-Scaffold)是由天然胶原蛋白制成的含有微米级孔洞的立体网状结构,主要用于干细胞放大培养,临床前体外药物测试,体外肿瘤模型培养,人造皮肤培养等。目前已经应用于细胞治疗、新药开发、精准医学等领域,有数十种不同类型细胞成功培养案例,且已发表大量高水平文献。
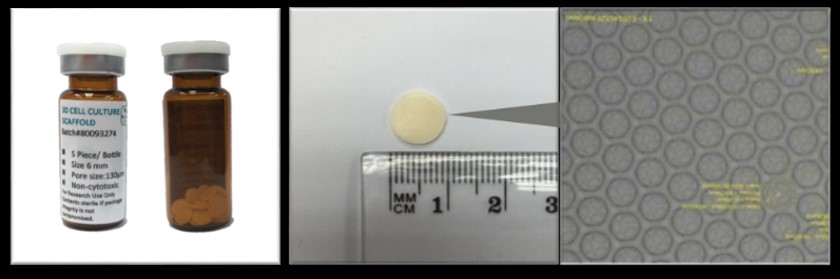
TanttiTM生医支架具有以下优势:
• 材质 – 支架材料采用胶原蛋白、蛋白聚糖、壳聚糖等天然高分子材料,可长时间培养细胞,仿生环境适于维持干细胞活性
• 细胞可回收 – 支架可用胰酶消化,实现细胞完全回收,满足更多实验需求
• 孔径 – 支架孔径大小均一,孔孔相连,结构批次稳定
• 注射型材料 – 天然高分子生物材料,可注射动物
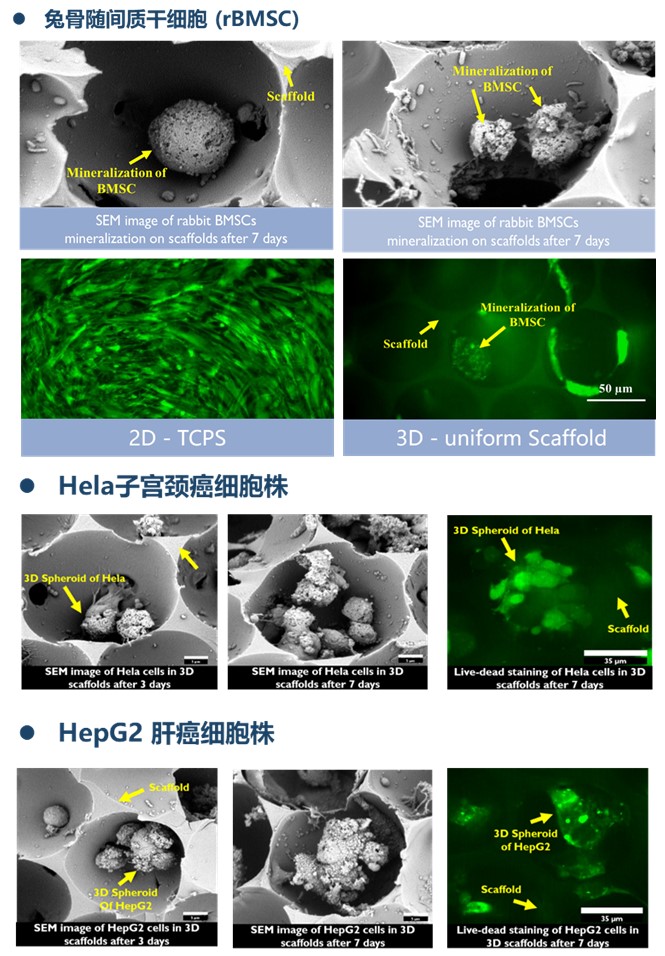
TanttiTM生医支架使用方法:
细胞培养
• 将支架分别放入 48 well (或 96 well) 孔板中 6®6
• 细胞稀释成0.5~5 x 106 / ml悬液加入孔板支架上,48孔板加入80μl/孔(或96孔板加入40μl/孔),如右图
• (可选) 将接种细胞的支架放入37 ℃、5% CO2细胞培养箱孵育10~15min
• 将新鲜培养基沿孔边缘缓慢加入,然后将细胞放入培养箱。培养基添加量:48 孔板 :1000 ul/孔;96 孔板:200 ul /孔
• (可选) 培养过夜后,将3D支架转移至新的孔板内,重新添加培养基继续培养
• 按照一般细胞培养流程,定期更换培养液
支架溶解
• 3D支架用 1 x PBS 泡洗三次 (5~10min / 次)
• 将支架放入2ml 离心管内
• 加入37 ℃ 预热的 0.25% 胰酶 (支架必须完全浸泡于胰酶溶液中)
• 涡旋混合均匀 3~5秒后,1500 rpm室温 (或37 ℃) 离心 5 分钟,即可溶解支架
生医支架(Bio-Scaffold)规格如下表,除了可以满足常规96/48孔板内细胞3D培养之外,还可用于生物反应器进行干细胞放大培养。
|
孔径
|
直径
|
规格
|
GSB110625
|
75 µm
|
For 96 well
|
25 pieces / vial
|
GSB110650
|
75 µm
|
For 96 well
|
50 pieces / vial
|
GSB120625
|
150 µm
|
For 96 well
|
25 pieces / vial
|
GSB120650
|
150 µm
|
For 96 well
|
50 pieces / vial
|
GSB130625
|
300 µm
|
For 96 well
|
25 pieces / vial
|
GSB130650
|
300 µm
|
For 96 well
|
50 pieces / vial
|
GSB110925
|
75 µm
|
For 48 well
|
25 pieces / vial
|
GSB110950
|
75 µm
|
For 48 well
|
50 pieces / vial
|
GSB120925
|
150 µm
|
For 48 well
|
25 pieces / vial
|
GSB120950
|
150 µm
|
For 48 well
|
50 pieces / vial
|
GSB130925
|
300 µm
|
For 48 well
|
25 pieces / vial
|
GSB130950
|
300 µm
|
For 48 well
|
50 pieces / vial
|
GSP110696
|
75 µm
|
For 96 well
|
96 pieces / 96 well plate
|
GSP120696
|
150 µm
|
For 96 well
|
96 pieces / 96 well plate
|
GSP130696
|
300 µm
|
For 96 well
|
96 pieces / 96 well plate
|
GSP110948
|
75 µm
|
For 48 well
|
48 pieces / 48 well plate
|
GSP120948
|
150 µm
|
For 48 well
|
48 pieces / 48 well plate
|
GSP130948
|
300 µm
|
For 48 well
|
48 pieces / 48 well plate
|
TanttiTM生医支架已发表文献:
· Lee J., et al., Inverted colloidal crystal as three-dimensional microenvironment for cellular co-cultures. Journal of Materials Chemistry (Impact factor: 6.626), 2006, 16: p.3558-3564.
· Peyton S. R., et al., Marrow-derived stem cell motility in 3D synthetic scaffold is governed by geometry along with adhesivity and stiffness. Biotechnol Bioeng (Impact factor: 4.243), 2011, 108(5): p. 1181-1193.
· Lee J., et al., Engineering liver tissue spheroids with inverted colloidal crystal scaffolds. Biomaterials (Impact factor: 8.387), 2009, 30(27): p. 4687-4694.
· Madden L. R., et al., Proangiogenic scaffolds as functional templates for cardiac tissue engineering. Proceedings of the National Academy of Sciences of the United States of America (Impact factor: 9.432), 2010, 107(34): p. 15211-15216
· Osathanon T., et al., Microporous nanofibrous fibrin-based scaffolds for bone tissue engineering. Biomaterials (Impact factor: 8.387), 2008, 29(30): p. 4091-4099.
· Kuo Y. C. and Tsai Y. T., Inverted colloidal crystal scaffolds for uniform cartilage regeneration. Biomacromolecules (Impact factor: 5.583), 2010. 11(3): p. 731-739.